This paper presents the results of an expert workshop to identify key factors critical to expanding negative emissions technology deployment in Canada.
Patrick Shorey, Grace Awuor Arwa, Kristen R Schell and Ahmed Abdulla*
Published 17 September 2024 • © 2024 The Author(s). Published by IOP Publishing Ltd
, , Citation Patrick Shorey et al 2024 Environ. Res. Lett. 19 104076DOI 10.1088/1748-9326/ad786d
Article metrics
155 Total downloads
Submit
Abstract
Pre-empting the worst consequences of climate change requires both mitigation of emissions from the global energy system and carbon dioxide removal through negative emissions technologies. Despite their nascence, negative emissions technologies are being incorporated into nationally determined contributions to achieve ambitious targets. It is therefore urgent to build a scaffolding that enables their expansion. Here, we report results from a workshop that brought together 34 prominent stakeholders, including scientists, engineers, energy system analysts, economists, experts in public policy, and policy makers. Participants discussed the likely cost and performance of these technologies; elucidated the opportunities and risks facing deployment; and envisioned how nations might build the necessary scaffolding for expansion. The majority narrative is that negative emissions technologies will have a bridging role in decarbonizing existing assets. Different models of deployment were proposed. Reaching the scale of deployment necessary to meet emissions targets is lengthy and expensive. Financial and regulatory risks are seen as greater barriers to deployment at scale than technological risk. Greater certainty regarding carbon pricing, production tax credits, and support for geological characterization and trunkline construction could reduce the former. Critical to expansion is a large-scale increase in low-carbon power production; the implementation of regulatory frameworks that remove uncertainty surrounding investment decisions; and prudent societal engagement.
Export citation and abstract BibTeX RIS

Original content from this work may be used under the terms of the Creative Commons Attribution 4.0 license. Any further distribution of this work must maintain attribution to the author(s) and the title of the work, journal citation and DOI.
1. Introduction
Despite rapid recent growth in the deployment of low-carbon energy generation technologies [1], integrated assessment models (IAMs) of the global climate and energy system demand even more aggressive deployment rates in order to deeply decarbonize the global energy system by mid-century. Across jurisdictions, achievement of this target is lagging ambition, forcing analysts and policy makers to explore negative emissions technologies (NETs)–a suite of natural and engineered solutions that actively remove carbon dioxide (CO2) from the atmosphere. Even where emissions targets are met, NETs may be the most efficient way of mitigating hard-to-abate emissions such as from cement, steel, or aviation. Two such engineering solutions are direct air capture (DAC) and carbon capture and storage (CCS). Within the NETs literature, DAC refers to the removal of CO2 from the atmosphere at dilute concentration, while CCS refers to the capture of CO2 at higher concentrations at the point of emission.
NETs can stabilize the climate at targets enshrined in the Paris Agreement while the inertia-laden global energy system reorients towards low-carbon sources of energy generation. In fact, a growing number of emissions trajectories in IAMs envision the simultaneous deployment of large amounts of low-carbon technologies and NETs in order to achieve climate targets [2]. The potential need for significant amounts of NETs has stimulated both research and policy attention, with policy makers exploring the role that different NETs might play in their decarbonization plans. For example, Canada is modelling a range of approaches for achieving its legislated net-zero emission target. In its long-term strategy submission to the United Nations Framework Convention on Climate Change (UNFCCC), most of these approaches involve potentially significant DAC deployment. Several scenarios envision upper bounds of 200 Megatonnes of CO2 (MtCO2) removal per year through DAC by 2050 [3].
Canada is a geographically large, resource-intensive, and industrialized country. At the same time, it has a relatively small population, a harsh climate, and limited connectivity in its electric power infrastructure (across both latitudinal and longitudinal axes). These features increase the resource intensity of some hard-to-abate sectors like heavy-duty freight, aviation, and industry, and have increased attention paid to carbon capture nationwide. However, the fossil energy industry is concentrated in the Western provinces of Alberta, British Columbia, and Saskatchewan: it is these three provinces (and especially the first) that have devoted most effort to characterizing carbon storage potentials. Although carbon storage capacity is known to exist elsewhere, including in Ontario and Atlantic Canada, the efforts (and expertise) devoted to these formations has been much lower.
The science and engineering of DAC is a topic of active and multifaceted research; a systematic literature review of these is beyond the scope of this paper. Relevant for this letter is the recent discussion surrounding the importance of policy in deploying DAC. For example, Shayegh et al have identified that ‘policy and regulations’, rather than cost, social acceptability, or technical challenges, will be the most important factor in limiting future DAC deployment [4], recognizing that policy and regulatory barriers influence and overlap with myriad economic and social concerns. Policy makers keen on developing additional, targeted policy signals for investment in DAC must consider what stakeholders in industry, academia, and policy making identify as key development and deployment bottlenecks, as well as actions that must be taken for future expansion to be achieved–assuming that future expansion is a goal.
This workshop adds additional context and a Canadian perspective to recent studies of carbon dioxide removal (CDR) and NETs policies within Europe [5] and the OECD [6]. Yang et al [5] surveyed NETs project developers and financiers within Europe and found that the greatest barriers to DAC were seen as lack of inherent demand, high energy consumption, access to finance, and lack of long-term policy certainty. Regulatory risk, public acceptance, and resource limitations were seen as less important. Policy preferences were also collected. Schenuit et al [6] analyzed CDR policy from nine OECD cases, and found they could group integration of CDR into climate policy in three approaches; (1) incremental modification, (2) early integration and fungibility, and (3) proactive CDR entrepreneurship. In addition, Hanna et al [7] modelled an emergency deployment of DAC and found that costs were less important than scaling capacity and learning rates, noting that even very large amounts of DAC capacity require concurrent emissions reductions.
Here, we report the results of a two-day, invitational, structured workshop that was organized in Ottawa, Ontario, Canada on May 30 and 31, 2023. The workshop brought together 34 prominent stakeholders, predominantly from Canada and the U.S., including scientists, engineers, energy system analysts, economists, experts in public policy, and policy makers. Emphasis was made on ensuring that representatives from Canada’s diverse provinces were included. The goals of the workshop were to: (1) discuss the likely cost and deployment potential of DAC and CCS in both the near-term and long-term; (2) elucidate on the opportunities and risks facing their deployment; and (3) envision how Canada and similar nations might build the necessary scaffolding that enables expansion. The paper proceeds as follows: section 2 presents the main methods employed to extract participants’ preferences or judgments. Section 3 presents key results from the workshop; section 4 elaborates on these results by providing additional context for the responses. Section 5 provides concluding thoughts.
2. Methods
The workshop was divided into eight sessions. The first was dedicated to outlining proposed (including modelled) net-zero emission pathways for the Canadian economy, and the role that NETs play in them (figure 1, Supplementary Information (SI) figures S1–S4). Session two listed, discussed, and ranked project features that would impact the likelihood of successful deployment of DAC and CCS projects in Canada–this session leveraged the presence of industry veterans to determine how future projects should be structured to enhance the prospects of success (SI figures S5–S6). Session three focused on CO2 utilization options in the short (2035) and long (2050) term (SI figure S7). This session included extensive discussion of the regulatory framework governing capture and storage of CO2 in different parts of Canada, including actions that must be pursued in the near-term to encourage long-term success. The next two sessions were devoted to research and development (R&D) on DAC and CCS–specifically, where the largest improvements in cost and performance might materialize (figure 3, SI figures S8–S10). Discussions regarding solvent and sorbent capture potentials, energy requirements, and system integration challenges occurred within these sessions.
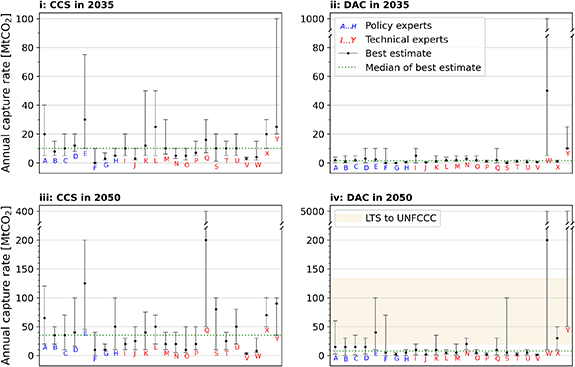
Figure 1. Lower bound, best, and upper bound estimates of net CO2 capture by CCS and DAC in Canada in 2035 and 2050. The pale yellow band in subpanel (iv) represents ECCC modelling of the emissions reductions attributed to DAC by 2050 under the ‘current assumptions scenario’ within Canada’s Long-term Strategy Submission (LTS) to the UNFCCC [11]. Note, in (iv), the best estimate of participant Y is 500 MtCO2e.
Download figure:
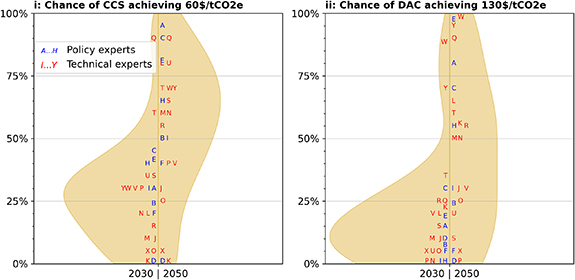
Figure 2. Likelihood of achieving targeted cost reductions for (i) CCS and (ii) DAC by 2030 and 2050.
Download figure:
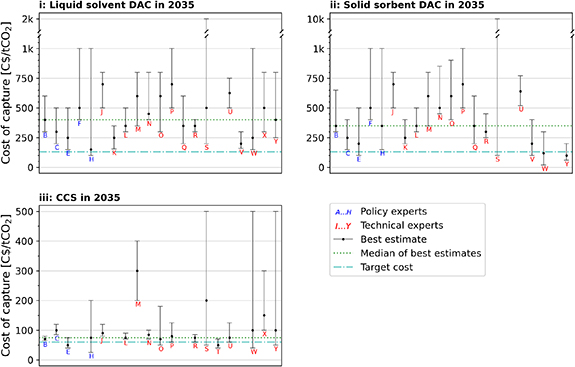
Figure 3. Predicted ranges of the cost of net carbon removal in 2035 by technology: (i) liquid solvent DAC; (ii) solid sorbent DAC; and (iii) CCS.
Download figure:
The second day–the remaining three sessions–was devoted to policy making: participants co-developed policy packages to accelerate NET deployment (SI figure S11) and articulated how Canada might build a scaffolding for deployment (figures 4 and 5). This included discussion of: (1) no-regret actions that we could take now to enhance the likelihood of future NET expansion; (2) bottlenecks that must be resolved over the next 5–10 years; and (3) developments over the next 5–10 years that could make or break NET deployment (figure 6). The last question is critical because it centers risks and forces participants to think in failure space.
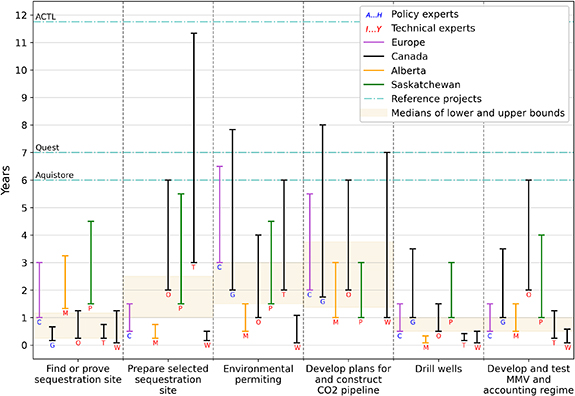
Figure 4. Assessments of the time required to pass key clearance points in the development of a new carbon capture site across different jurisdictions. Dashed teal lines represent the time taken to commission three carbon management projects in Canada: Aquistore, Quest, and the ACTL.
Download figure:
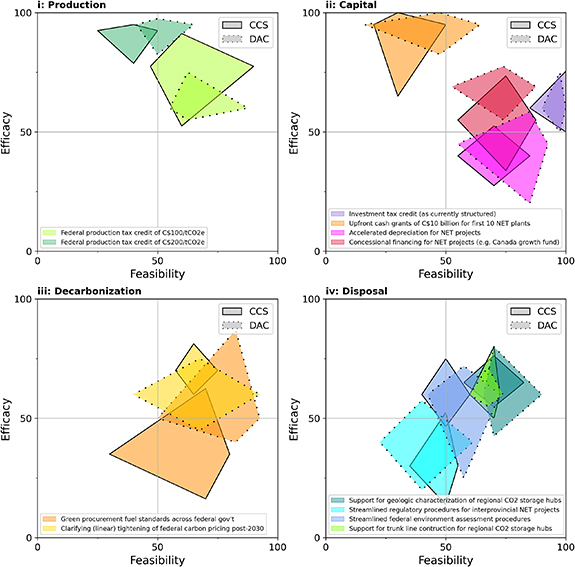
Figure 5. Assessments of the efficacy and feasibility of different: (i) production, (ii) capital, (iii) decarbonization, and (iv) CO2 disposal incentives that could be provided by the Federal Government. Policy incentives were ranked ordinally then weighted, with polygon limits defined by the interquartile ranges, centered on the median score. In the workbook, we defined efficacy as credibility to a developer, and feasibility as political feasibility.
Download figure:
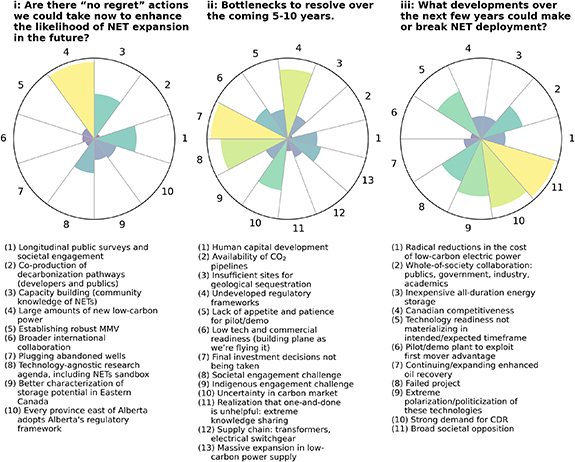
Figure 6. Assessments of (i) ‘no regret’ actions that Canada can take to enhance NET expansion; (ii) bottlenecks that need resolving; and (iii) near-term developments that could make or break NET deployment. Critical to NET expansion is a large-scale increase in low-carbon power production; the implementation of regulatory frameworks that remove uncertainty surrounding investment decisions; and prudent societal engagement.
Download figure:
Throughout, participants recorded their response to a variety of exercises in personal workbooks: the workbook is reproduced in the SI as appendix A, along with additional results. Workbooks were anonymized, and individual respondents are identified here by a unique letter. Exercises included chip allocation, rank-and-weight, linear ranking, pair-wise ranking, Likert scales, and open-ended quantitative. Descriptions of each exercise are given in the workbook. At the beginning of the workshop, participants ascribed a range of percentages corresponding to likelihood phrases used in the Likert scales (SI table S1). Most questions included the option for written feedback, and some allowed participants to add attributes felt to be missing. Participants were categorized by the authors as being either policy or technical experts. These are denoted in the figures by marker colour, with policy experts in blue and technical experts in red.
3. Results
Broadly, discussions at the workshop can be divided into three categories. The first is the expected role that CCS and DAC are likely to play in the transition to a low-carbon energy system in both the near-term and the longer-term (2050); the latter target was enshrined into Federal Canadian law in 2021 [8]. Notably, the median participant believed it unlikely that Canada would achieve a 40% reduction in emissions by 2030 (note, all % emissions reductions are with respect to 2005 levels), and somewhat unlikely that Canada would achieve net-zero emissions by 2050. For a distribution of the responses see SI figure S1. That policy experts were unconvinced of prominent DAC expansion after learning of its proposed role in Canada’s long-term submission to the UNFCCC is both interesting and concerning. The weak confidence in DAC deployment in Canada (and potentially elsewhere) merits further investigation since, increasingly, the deployment of CDR is crucial to achieving proposed net-zero goals which are enshrined in legislation in some jurisdictions.
Second is the expected cost and deployment timelines of NETs–these are key to policy development and making final investment decisions. Third is a forward-looking investigation of deployment potential: what are the research priorities and policy instruments that could scale up NET deployment, and what changes in the broader energy system provide opportunities or pose challenges to NET deployment. We present results from each of these three categories in turn.
3.1. The expected role of DAC and CCS in the energy transition
In meeting the 2050 goal of net-zero emissions, 70% of participants believed CCS should play a major role (5% of emissions reductions), rather than a minor role, while participants were almost evenly divided on whether DAC should play a major or minor role, see SI figure S3. Participants were also asked to elaborate on their responses to this normative question; major themes from these written responses are summarized below.
The general sentiment was that CCS should be used as an interim measure to decarbonize existing generation and heavy industry, while low-carbon generation expansion and electrification of industry proceed. In the long-term, CCS has a role in capturing emissions from hard-to-abate industries such as cement, steel, and remaining oil refineries. There was a range of opinions on long-term use of CCS for fossil fuel generation. Some participants thought that this should be avoided at all costs, due to uncertainty related to geological storage, while others saw CCS as having an appropriate long-term role for jurisdictions with low renewable capacity.
It was recognized that DAC has become a necessity, despite its inherent challenges, due to emissions overshoot of the global carbon budget. DAC is seen as having a long-term role mitigating hard-to-abate, dilute, decentralized, or fugitive emissions, such as from agriculture or aviation. DAC could have an expanded role in the latter half of the century, as atmospheric CO2 concentrations accumulate, and even radical emissions reductions are unable to meet warming goals. As such, participants supported DAC capacity building. However, some participants only supported a role for DAC in addressing historical emissions in Canada; i.e., DAC should not enable continued burning of fossil fuels [9].
Best estimates of annual capture rates by 2035 for CCS range from 0 to 30 MtCO, with a median estimate of 10 MtCO. By 2050, the range of best estimates is from 5 to 200 MtCO, with a median of 35 MtCO, see figure 1, sub-panels i and iii. Note that Canada captured approximately 3 MtCO from point sources of greenhouse gas emissions in 2022 [10]. Estimates of no capture from CCS in 2035 reflect an expectation that carbon capture amounts will decrease in the near-term.
Predictions for DAC are generally lower, with the median best estimate across respondents being 1.5 MtCO by 2035 and 7.5 MtCO by 2050. There are, however, outliers who predict large amounts of capture by 2050–200 or 500 MtCO, see figure 1, sub-panels ii and iv. In comparison, for Canada to meet its net-zero targets, modelling by Environment and Climate Change Canada (ECCC) finds the need for between 0 and 201 MtCO of DAC capture capacity by 2050, depending on the scenario, with middle values between 44.5 and 84.5 MtCO [11].
3.2. Expected costs and time to develop sequestration sites
Estimates of the costs of NETs that are published in the literature include a range of 130-321 CAD/tCO for liquid solvent DAC [12] and 65 USD/tCO for CCS [13]. We acknowledge that cost estimates for DAC are highly uncertain: recent comparable estimates give a range of 275-494 CAD/tCO2e [14]. Participants were asked how likely these technologies are to achieve the lower limit of these estimates by 2030 and by 2050, see figure 2. These cost targets were seen as unrealistic by 2030 but achievable by 2050 for CCS. There was substantial uncertainty in the long-term cost of DAC.
Participants were also asked to provide lower bound, best, and upper bound estimates on the price of avoidance or removal in 2035 for CCS, liquid solvent DAC, and solid sorbent DAC (see figure 3). Liquid solvent and solid sorbent DAC had similar estimates from most participants, with a median best estimate of 400 and 350 CAD/tCO, respectively. Estimates for CCS have much lower variability, with a median best estimate of 80 CAD/tCO, in closer agreement with the reference price of 60 CAD/tCO than DAC. This is not unexpected, as CCS is a more mature technology that saw approximately 3 MtCO2e of gross capture in 2022 in Canada [10].
As for achieving cost reductions in both DAC and CCS systems, workshop participants stressed the importance of investing in efforts to reduce variable operating costs first (primarily energy costs), followed by reducing capital costs and improving regulatory processes. The importance of improved project management and reduced labour costs were also highlighted (see SI figure S10).
Participants predict that deep geologic storage and enhanced oil recovery (EOR) will comprise the majority of CO2 sequestration capacity for Canadian projects in the near-term. By 2050, the largest share of sequestration capacity will be for deep geologic storage (see SI figure S7).
Because the participants included regulators, policy makers, and investors with experience in deploying CCS projects in the real world, judgments were elicited on the time required to prepare a sequestration site for injection of captured CO2, including the development of pipeline infrastructure. First, a list of key project steps were co-produced by workshop participants as a group. Then, participants estimated the duration (as a range) for each of these key project steps. Results are presented in figure 4. Note, participants were initially asked about the time to resolve legal challenges as well; these are not shown, as respondents argued that legal delays are too variable to be amenable to a general estimate. It is further noted that some of these steps can be completed in parallel. The longest step identified was to develop plans for and construct CO2 pipeline (medians of 1.5–4 years), followed by environmental permitting (medians of 1.5–3 years) and prepare selected sequestration site (medians of 1–2.5 years), with the remaining steps taking one year or less. There was considerable variation outside of these median ranges.
The total development time for three Canadian projects is included for comparison; these times are based on publicly available documentation and include time from the first public consultations (if reported) to commercial injection. The Alberta Carbon Trunkline (ACTL) is a 240 km CO2 pipeline in Alberta, associated with three separate capture facilities [15]. Quest is a Shell CCS project including 84 km of pipeline, also in Alberta [16]. Aquistore is the geological storage and 2 km pipeline serving SaskPower’s Boundary Dam Carbon Capture Facility (currently operating with a research permit) in Saskatchewan [17, 18].
Summing the median lower and upper bounds of the six steps (i.e. the yellow shaded ranges in figure 4) identified gives a range of 5.1–12.4 years, which is similar to the range represented by the three reference projects, as well as recent estimates for sequestration projects in the U.S. [19]
The workshop discussed the state of carbon capture and storage regulations across provinces. A summary of Alberta’s modifications to its regulatory practices, including the Carbon Capture and Storage Statutes Amendment Act [20] and the Carbon Sequestration Tenure Regulation [21], illustrate how licensing and regulation of projects can be streamlined by resolving questions of access to pore space, the levels of monitoring and verification that are required, and the question of long-term liability for captured carbon. Experts from other provinces expressed interest in adapting some of Alberta’s procedures, though they stressed the need for much greater technical expertise at the regulatory level, so that government is able to license and monitor credible projects while safeguarding public health and the environment.
3.3. Forward-looking assessments of deployment potential
Participants provided their assessments of how best to scale up DAC deployment, answering questions regarding research priorities and features of successful project development. Insofar as successful upscaling of NETs is concerned, they also provided: assessments of the most effective policies to accomplish that goal; no-regret actions that Canada must undertake; bottlenecks to NET deployment; and actions over the coming 5–10 years that could make or break NET deployment. This section provides a summary of participant responses to all six questions.
Research priorities. R&D to enhance solid sorbent systems should focus on developing novel sorbents, specifically with respect to increasing sorbent lifetime and reducing the temperature, and thus thermal energy requirements, of desorption (see SI figure S8, sub-panel i and ii). In contrast, R&D to enhance liquid solvent systems should focus on heat transfer and integration, followed by contactor and then calciner design (see SI figure S8, sub-panel iii).
Features of successful project development. Through a chip-allocation exercise, participants ranked project features according to their importance to the successful development of a project (with the option to write-in additional features). The full list of features, including rationale for inclusion, are given in the SI appendix A. Ordered rankings, including per-expert weighted scores, are shown in SI figure S5.
Overall, there was a group of three features that were ranked higher on average than most others, a group of five in the middle, and a group of six that ranked lower. The highest ranking features are technology agnostic, focusing on financial and regulatory uncertainty. They include: (i) credibility of revenues, (ii) credibility of incentives, and (iii) regulatory challenges. This is in contrast to the European context [5], where regulatory uncertainty was seen as less of a barrier than policy uncertainty. The middle-ranked features are mostly concerned with characteristics of the technology in question, and include: (i) public opposition, (ii) technological readiness level, (iii) burden of CO2 disposal, (iv) capital cost, and (v) population proximity. Lastly, the majority of the lowest ranked features are project specific, and include: (i) institutional setting, (ii) project execution, (iii) employment impacts, (iv) stakeholder misalignment, (v) plant siting, and (vi) proximity to disadvantaged communities.
Many participants emphasized the importance of the environmental impact of the project, appropriate engagement with Indigenous communities, as well as availability of low-carbon energy. These features were not included in the rankings as not all participants ranked them.
Policy design. Participants were asked to rank and weight 12 different federal policy packages according to their feasibility and efficacy in incentivizing NET deployment. The polygons in figure 5 are defined by the 25th and 75th percentile of the responses, centered on the median (sub-panels represent policy categories), so that smaller polygons represent more agreement between participants. Following the schema articulated by Njuguna [22], the top right quadrant represents policy incentives that should be acted upon, the top left are effective but require buy-in to become feasible, the bottom-right need modification to be more effective, and the bottom left, neither effective nor feasible, require a re-design. While this exercise had participants focus on government incentives, in section 4 we discuss how some participants resented providing such support because it is not in the interest of taxpayers.
Recommended policy incentives include (i) federal production tax credit of C$100/tCO2e, (ii) investment tax credits, (iii) linear tightening of federal carbon pricing post-2030, (iv) support for geological characterization of regional CO2 storage hubs, and (v) support for trunk line construction for regional CO2 storage hubs. Production and capital incentives were deemed somewhat more effective; some economy-wide incentives for decarbonization (that would also help expand NETs) achieve high levels of effectiveness and feasibility. These policy packages do not represent the full range of NETs policy options under consideration by various jurisdictions, for example, where fossil fuel suppliers are required to invest in NETs to access fuel markets.
No regret actions, bottlenecks, and make-or-break developments. Participants brainstormed responses to the following three questions: (i) Are there ‘no regret’ actions we could take now to enhance the likelihood of NET expansion in the future? (ii) What are the bottlenecks to resolve over the coming 5–10 years?, and (iii) What developments over the next few years could make or break NET deployment. Participants then ranked their top 5 responses. The total relative scores of each response are shown in figure 6, after applying a linear weighting to the ranking.
4. Discussion
The format of the workshop enabled the extraction of individual assessments from participants, followed by discussion that elaborated on these assessments. Where no consensus existed, participants could be broadly divided into clusters. While this paper focuses on Canada, a systematic comparison of CCS and DAC policies across nations would be useful. For example, the US’ approach of deploying DAC hubs (and hubs for other technologies) aligns with some of the infrastructure creation policies discussed at the workshop. Similarly, Alberta’s regulatory approach to CCS saw much discussion, including how the province dealt with controversial questions regarding access to pore space and long-term liability for stored CO2. Countries can learn from the Canadian experience, and Canada can undoubtedly learn from other nations’ strategies.
4.1. Disagreement between policy and technical experts
In some cases, policy and technical experts clearly disagreed in their assessments. For example, policy experts were much less likely to believe that Canada will achieve net-zero emissions by 2050 (figure S1). When it came to sources of emissions reductions (to meet 2030 goals), policy experts had higher expectations for light duty vehicles, but lower expectations for the agriculture sector, than technical experts (figure S2). Considering the role of NETs in Canada’s emissions reductions, while the majority of both groups of experts agreed that CCS should play a major role in achieving net-zero targets, policy experts’ belief that DAC should play a similarly major role were at odds with those of technical experts (figure S3). In contrast with this normative question, technical experts thought it more likely that DAC would contribute to emissions reductions by 2050 (figure S4). Lastly, when ranking the importance of NET project features, policy experts were less concerned about financial credibility and the burden of CO2 disposal, and slightly more concerned with plant siting and related equity concerns (figure S5), likely stemming from their acknowledgment of how challenging the deployment of large energy infrastructure is, and how large, ambitious efforts are often thwarted by poor project planning or dismissiveness of socio-political concerns.
4.2. Diversity of opinion regarding government’s role in advancing NET deployment
There was no consensus on the Federal Government’s appropriate role in advancing NETs. Instead, three distinct clusters emerged: those who opposed the provision of further support; those who supported extending to NETs traditional forms of government support, with more concentrated short-term support during the market creation phase; and those who called for a radical re-imagining of NETs deployment, with long-term intensive government support. Here, we briefly describe the attitudes of members of each cluster.
Those in opposition–including participants with long careers monitoring the natural resource sector in Canada–advocated passionately for no additional incentives or regulatory support (on the part of the Federal Government). ‘This industry is already over-subsidized’, was one refrain, and another was that there should be ‘no more paper’–as in, no more legislative or regulatory support. This cluster of participants was also deeply suspicious of other suggestions to accelerate NETs up-scaling, arguing that previous efforts at ‘knowledge sharing’ or the formation of deployment hubs had failed due to the commercial sensitivity that surrounds the precise design of energy facilities.
A second cluster of participants expected government to support NETs in a manner similar to other emission mitigation technologies, including energy generation infrastructure. These modes of support include upfront capital, various tax credits, and work that promotes and sustains a broader ecosystem that supports NETs.
The third cluster of participants supported some of these incentive types, but fundamentally disagreed with the notion that NETs must be promoted like other emission mitigation technologies. In their view, this cannot be considered a normal industrial enterprise. Rather, it is a large-scale waste management enterprise, like municipal solid waste, that must be publicly supported throughout.
4.3. Lack of political leadership and technical expertise threatens both economy and climate
Most participants agreed that there is an opportunity for Canada to lead in carbon capture technologies, including by exporting technology and carbon management expertise to the rest of the world, with some deeming this a ‘better way of addressing legacy emissions’ (i.e., historical emissions contributing to current atmospheric CO2 levels). At the same time, both technical and policy experts lamented Canada’s tepid approach to both NETs and decarbonization broadly; the lack of political leadership and direction at all levels of government; and the profound lack of technical expertise outside the provinces of Alberta and, to a smaller extent, Saskatchewan.
Among the concerns raised was a lack of awareness among policy makers and the public that industry runs on heat–and that a reconfiguration of industrial plants from thermochemical to electrochemical processes is a significant endeavor for which the nation is unprepared. A second concern raised by a smaller number of participants is decarbonization through deindustrialization in provinces that seek to meet net-zero emission targets while lacking technical expertise in carbon management. The nation’s manufacturing powerhouse, Ontario, was singled out as susceptible to this fate without urgent corrective action. Industrial electrification in Ontario is underway in some sectors like steel, but participants raised concerns about the fact that Ontario is pursuing large investments in the new economy–such as both electric vehicle (EV) and EV battery plants–that are both large sources of new demand and yield products that comprise additional sources of electricity demand.
Participants elaborated the clearance points required to commission a new project before assessing the length of time it would take to clear each. Those with expertise in CCS noted that prudent investors should preferentially pursue CCS projects that eliminate risks like legal challenges, federal environmental assessments, or public opposition. All participants, even those that recommended limited additional policy support for NETs, pointed to a growing sense of urgency regarding the 2050 goal. Finally, one participant from outside Canada noted that, in many European jurisdictions, policy makers cannot mention CCS without a backlash, reducing the range of options available to achieve deep decarbonization.
4.4. Environmental and socio-political concerns
While participants recognized the challenge of climate change mitigation, most cautioned that decisions regarding resource deployment are not trivial and tradeoffs between NETs and low-carbon energy projects will be made. This is driven not just by limited capital investment and policy ambition, but also by the sheer physical needs of NETs–primarily energy but also pipelines, storage capacity, and human capital. Although in competition for similar resources, both increased deployment of NETs and of low-carbon energy projects feature in most pathways to net-zero emissions.
Participants grappled with the uncertainties involved in expanding NETs: the deployment of these systems is driven by environmental concerns, and participants therefore deemed it likely that their environmental impacts will be top of mind. Since the only large-scale carbon removal projects currently being developed in Canada are CCS, it remains unclear whether scrutiny of environmental impacts will be more stringent for NETs than it has been in Canada for other large infrastructure projects.
A broader point repeated throughout the workshop is the importance of risk communication to any large-scale rollout: participants noted that the ‘trust in experts’ model is broken in Canada. Examples offered included waste incineration, nuclear technologies, failed wind projects, hydropower siting, and other energy infrastructure like pipelines and natural gas terminals. Participants stressed that these challenges are not insurmountable: there are several examples of successful project development which relied on successful Indigenous engagement models or practices. These concerns apply broadly to utility-scale energy infrastructure, and it is a testament to their importance that societal opposition and extreme polarization were ranked first and third on the list of developments that could ‘make or break’ NET deployment (figure 6, subpanel (iii)). The ‘societal engagement’ challenge was also ranked high as a bottleneck to large-scale deployment.
5. Conclusions
A workshop elicited the judgments of 34 participants on the future of NETs, with a focus on the Canadian context. The majority narrative is that NETs will have a bridging role in decarbonizing existing industrial and generation assets, and a long-term role in abating the most intractable emissions, including legacy emissions of CO2.
Reaching the scale of deployment necessary to meet emissions targets will be lengthy and expensive. Efforts to reduce cost should focus on reducing variable operating costs. Most captured CO2 is destined for geological storage, and development of a storage site alone is expected to take 5–12 years. Given the expected need for CCS and DAC in Canada’s long-term submission to the UNFCCC, failure to expand this capacity could, in its own right, risk the failure of Canada’s net-zero emission plans.
Despite DAC being a relatively immature technology, at this point financial and regulatory risks are seen as greater barriers to deployment at scale than technological risk. Developing vast amounts of low-carbon power is a no-regret action that governments can take now that enhances the likelihood of expansion. Recommended Canadian federal policy packages to improve NETs deployment include: investment tax credits, greater certainty regarding carbon pricing post-2030, a federal production tax credit of 100 CAD/tCO2e, concessional financing, and support for geological characterization and trunkline construction for regional CO2 storage hubs.
Acknowledgment
This research was undertaken with the financial support of the Government of Canada, and of Carleton University. The authors thank the 34 participants who attended the workshop.
Data availability statement
All data that support the findings of this study are included within the article (and any supplementary files).
Conflict of interest
The authors declare no competing interests.